Dark matter
Article curated by Ed Trollope
Dark matter is a proposed solution to a number of unsolved problems in physics. The name dark matter refers to material that does not emit or absorb light (this is why we cannot see it, hence dark) but can affect the universe on large scales due to gravitational interactions.
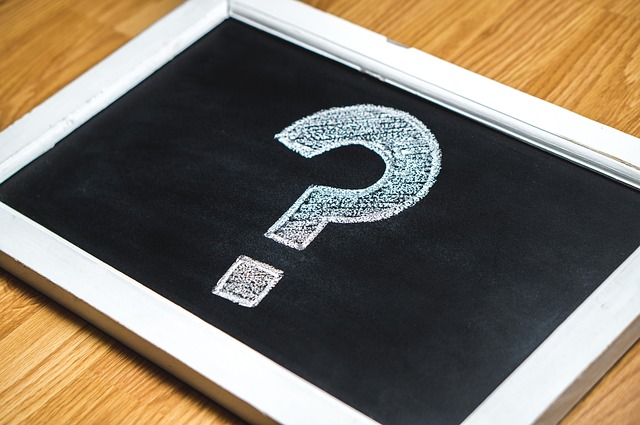
Spinning galaxies
As we understand it, the rotation rates of galaxies are related to their mass (the mass of their constituent stars, gas and dust). We can infer the mass of a galaxy based on what we can 'see' of it. However, when observing galaxies in the universe, stars near the outside of galaxies appear to be moving faster than can be accounted for by the gravitational attraction of the mass of stars and dust we are able to see in them.


On a larger scale, galaxies are organised into clusters: enormous collections of up to a thousand galaxies, bound by gravity. Again, the material we are able to detect directly indicates that the velocity of individual galaxies ought to decrease with distance from the cluster's centre. What we actually measure is that this value remains fairly constant.This indicates that our calculations of the galaxy's (or galaxy cluster's) mass based merely on what we can see are inaccurate, there must be something else that we cannot see.


The cosmic microwave background radiation is the afterglow of the big bang, a fossil record of the radiation from the very early universe, an image of the moment only a few hundred thousand years into the life of the universe when everything had cooled enough for atoms to form. When these atoms formed, there were far fewer unattached charged particles around for light to interact with, allowing it to propagate freely, and the universe ceased being effectively opaque to light. By using very sensitive detectors, scientists are able to measure variations in the faint level of this fossil microwave radiation across the entire sky.
In the universe before this point, for normal matter, the electromagnetic force would have played a far more important role than gravity because it is so much stronger. However, matter was mostly composed of a very hot plasma of particles, due to which the transfer of force over any significant distance would have been impossible as these electromagnetic forces would have cancelled out. Our measurements of this background radiation show small but significant local variations which are understood to be the source of the structure in the universe today. Without these small areas of material varying in density compared to it's surroundings, we would be unable to explain how the material in the universe had grouped together to form large structures like galaxies or galaxy clusters and the massive, near empty voids in between them. The question we are faced with is: how did these variations form?


How many dwarf galaxies are out there?
Dwarf galaxies are small galaxies containing a few billion stars (hundreds of times fewer than our own Milky Way). The most widely accepted model used to explain the expansion and history of the universe to this point, known as the lambda-CDM model, predicts that there should be many more dwarf galaxies in the cosmos than we have thus far observed. This is because the way cold dark matter clumps together is predicted by computer simulations to result in increasing numbers of objects at smaller scales. It is possible that there are more dwarf galaxies out there, but if not, then the model may need to be altered to explain their comparative rarity.
The difficulty in detecting these dwarf galaxies lies primarily in the fact that they are very faint objects. The imaging technology necessary for their direct observation is relatively new and so many more dwarf galaxies local to our own cosmic neighbourhood may be discovered in the near future.

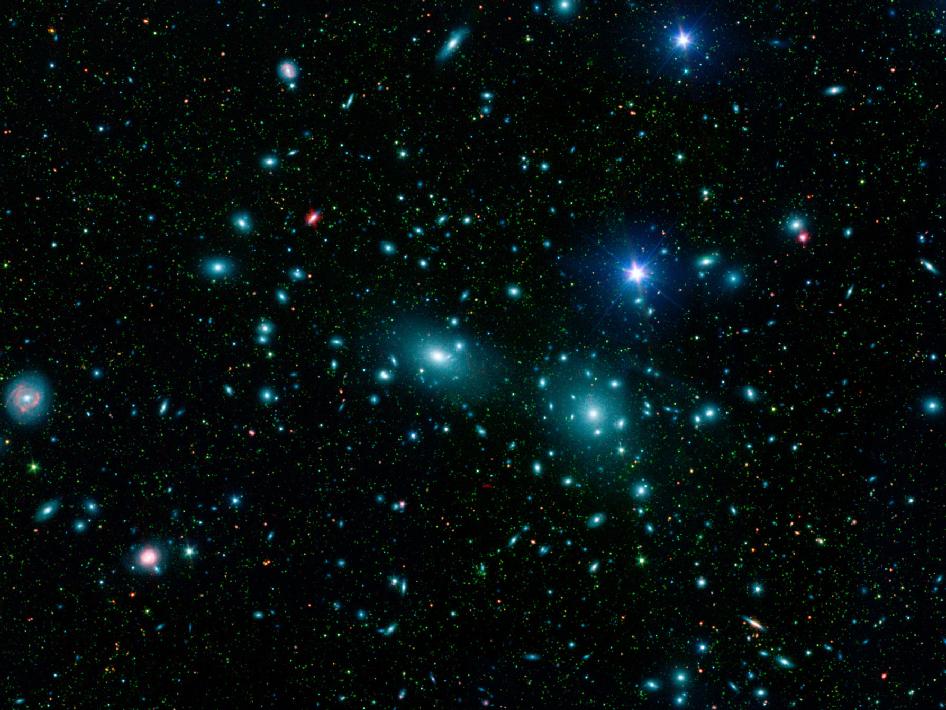
Smaller galaxies tend to be denser, and have a higher proportion of dark matter. Dwarf ellipticals may be amongst the most dark-matter dominated objects in the universe - and are the most common type of galaxy. But what role does dark matter play in the formation of galaxies, and why does it lead to these different proportions?

Cooking up Elements
The vast majority of the atoms we can detect in the universe were created very shortly after the big bang, in a process known as nucleosynthesis. Most of these atoms are Hydrogen and Helium, which formed in a specific ratio, along with some tiny amounts of Deuterium, Lithium, and Beryllium (heavier elements are created later in processes within stars). Calculations based on this ratio tell us that the density of the material making up these atoms was too low to account for the observed rate of expansion of the universe. This ratio has been measured closely by observing objects in the early universe. We are able to do this because the further away something we are looking at is, the further back in it's history we are seeing it, as light travels at a finite speed, and so takes time to reach us. Measurements of the expansion of the universe (by looking at how groups of galaxies are moving apart) have also been made. We can therefore ask where the mass responsible for this expansion rate comes from, given that it did not affect the ratio of elements created in the big bang.
Learn more about Big Bang Nucleosynthesis.


What's bending the light in the Bullet Cluster?
The path of a beam of light can be bent by the gravitational effect of an object’s mass. A sufficiently heavy object (for example a galaxy or cluster of galaxies) can act like a lens, bending light beams that pass close to it around a focal point, just like the lens in a telescope, or a pair of glasses. This is a process known as gravitational lensing. By observing the manner in which these objects bend the path of light from objects behind them, scientists can work out the point which this light is being bent around. By adding up the masses and locations of all the visible components of the galaxy cluster (stars, dust etc.) to obtain their centre of mass, we can work out the point this light ought to bend towards, but we see that it's actually that gravity is actually focusing this light around a different point[1]. So the natural question to ask is: what is pulling light towards this other point?

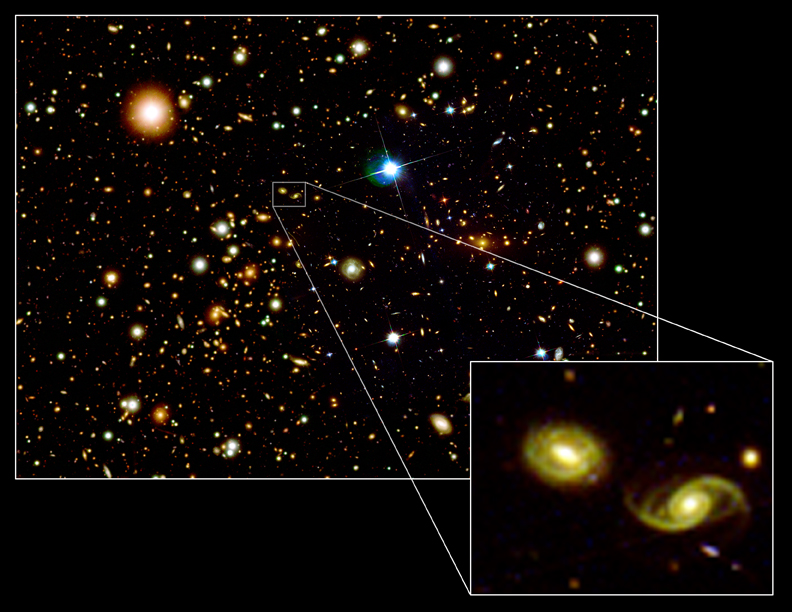
Dark matter has mass, but is not known to interact electromagnetically and so cannot be detected in the traditional way, with telescopes studying the electromagnetic spectrum (from radio, through visible light to X-rays). The gravitational effect of this mass could be responsible for the higher speeds of stars within galaxies, and of the galaxies within clusters. If this dark matter is indeed unaffected by electromagnetic forces, it may have been able to form structure in the universe before atoms had formed, this hypothesis could explain the variations observed in the cosmic microwave background. Its mass could also explain the observed expansion of the universe as well as the observed relative abundance of elements created in the period shortly after the big bang. Perhaps the most compelling evidence for the existence of dark matter however, is the gravitational lensing observed in the Bullet Cluster, this seems to show directly that mass is concentrated in an area other than ordinary matter, and cannot easily be explained in any other way (such as modifications to our understanding of the way gravity works).
Learn more about Evidence for dark matter.

So what is it?
There are a number of theoretical candidates for dark matter (a list which may well have more ideas added to it in the future) that could explain the apparent observed mass. Earth-based detectors may be beginning to see the first hints of interaction with some of these, however the precise nature of this material is exceptionally difficult to determine.
Determining the nature of dark matter is challenging in part because it is incredibly hard to isolate interaction events that may be occurring between dark matter and ordinary matter in order to study these further. Another factor in the difficulty facing research in this area is that our detection techniques are based to a large extent on assumptions, specifically about mass, and that dark matter might interact via the weak nuclear force. If these assumptions are false, scientists may be looking in entirely the wrong places.

The way in which stars are grouped in galaxies, galaxies in clusters and so on could be helpful to us in understanding Dark matter. This “hierarchy of structure” in the universe may offer some clues as to properties of dark matter, such as the mass of the particles responsible. This is because their mass would affect the speed at which they move compared to the speed of light, which in turn affects how structure would have formed in the early universe.

One theory, known as Supersymmetry, predicts that the particles we know of ought to have partners, which may be much heavier and which might make up dark matter. If dark matter is composed of these then it should be possible to detect them in particle accelerators on Earth. Mass and energy are equivalent, (using Einstein's famous e=mc2) so if these supersymmetric partners do exist, then the lightest of them are at least as heavy as the limit of the energy in these experiments. Although these become less likely as the energy level these accelerators can reach gets higher, we cannot rule them out completely.


Evolution of the Universe
One of the biggest questions in astronomy is how did the universe end up the way it is?
Clues lie in the formation of stars in nebulae, looking at galaxies of all ages across the universe, and studying the cosmic microwave background. Black holes may also hold clues - but did they form as the first stars did, or did the first stars form the first black holes?
Dark matter is also a key player in answering this question since most of the universe is believed to be made up of Dark matter and dark energy - but how can we detect these things and observe them, when they do not emit any kind of radiation or interact that strongly with matter?
Learn more about Evolution of the Universe.

Has dark matter been detected?
Several physicists from Johns Hopkins University have suggested that a black hole binary detected by LIGO could actually be considered a signature of dark matter
[2].
In a paper published in The Astrophysical Review Letters, Kashlinsky indicates that primordial black holes being dark matter would offer an explanation of observed cosmic infrared background fluctuations that cannot be accounted for by known galaxy populations
[3].
Despite arguments that cosmic microwave background constraints exclude primordial black holes in the mass range discussed, the researchers propose that due to the complex modelling required by the constraints, a significant (and difficult to quantify) uncertainty should therefore be associated with this upper limit
[2]. This means that the black holes observed by LIGO could potentially be primordial black hole dark matter[2].
In other words, the black hole binary detected by LIGO might be a primordial black hole, and primordial black holes could be dark matter; therefore, it is possible that LIGO may have detected dark matter, but is far from confirmed.


Another candidate for a possible detection of dark matter is the Hubble telescope, which may have discovered a dark matter core left behind after many galaxy clusters merged and collided 2.4 billion light years away, in a place called abell 520. Astronomers expected normal matter in galaxies and galaxy clusters to tag along with dark matter "like a dog on a leash", however observations have revealed what appears to be a 'dark core' containing far fewer galaxies than expected if galaxies and dark matter were anchored together.
The original observation was made in 2007, but no one probed further as the data was fairly poor and it did not lie with what astronomers believed based on observations of the bullet cluster. In the case of the bullet cluster, galaxies collided and dark matter and galaxies remained tied together. We know the dark matter is there, along with lots of hot x-ray emitting gas because of gravitational lensing effects. More recent data from the Hubble telescope confirmed what was found in 2007.
Some theories state that there are different types of dark matter, with some types being 'sticky' and interacting with itself. Another explanation is that the Abell 520 cluster merger was more complicated than the bullet cluster - which only contained two colliding systems. A third is that the galaxies are there, but are too dim to be detected yet. The next step now is to create a computer simulation to reenact the situation to see if anything more can be revealed.
We know of maybe six examples of high-speed galaxy cluster collisions where the dark matter has been mapped,
astronomer James Jee of university of California said. But the Bullet Cluster and Abell 520 are the two that show the clearest evidence of recent mergers, and they are inconsistent with each other. No single theory explains the different behavior of dark matter in those two collisions. We need more examples.
Furthermore, a dark matter core is only one possible explanation for what is being observed.

This article was written by the Things We Don’t Know editorial team, with contributions from DLR, Ed Trollope, Jon Cheyne, Grace Mason-Jarrett, and Alice Wayne.
This article was first published on 2015-08-27 and was last updated on 2017-05-21.
References
why don’t all references have links?
[1] Clowe, D., et al., (2006) A Direct Empirical Proof of the Existence of Dark Matter The Astrophysical Journal 648:L109-L113 DOI: 10.1086/508162
[2] Bird, S., et al., (2016) Did LIGO Detect Dark Matter? Physical Review Letters 116(20) DOI: 10.1103/PhysRevLett.116.201301
[3] Kashlinsky, A., (2016) LIGO GRAVITATIONAL WAVE DETECTION, PRIMORDIAL BLACK HOLES, AND THE NEAR-IR COSMIC INFRARED BACKGROUND ANISOTROPIES The Astrophysical Journal 823(2):L25- DOI: 10.3847/2041-8205/823/2/L25
Blog posts about dark matter

Recent dark matter News
Get customised news updates on your homepage by subscribing to articles